Introduction of Akihiro Goto's research
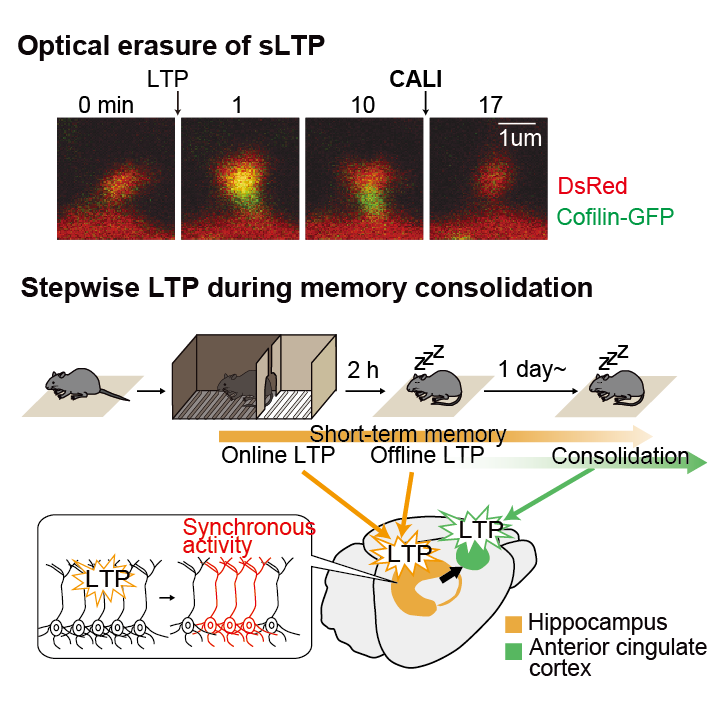
Analysis of cellular mechanisms of long-term storage of memory by new optogenetics (~2021)
Goto A, Bota A, Miya K, Wang J, Tsukamoto S, Jiang X, Hirai D, Murayama M, Matsuda T, McHugh T, Nagai T, and Hayashi Y.
Stepwise synaptic plasticity events drive the early phase of memory consolidation.
Science 374(6569) 857-863, 2021.
Memory Consolidation
When and where are memories formed and stored in the brain? This is an important topic in neuroscience. Long-term memory storage is also an essential function of our lives. Long-term and short-term memories are thought to be stored in different areas of the brain, as in the famous case of H.M. H.M., who had his hippocampus removed to alleviate seizures, retained his childhood memories but could not recall memories immediately before the surgery. This case suggests that post-learning memories are temporarily stored in the hippocampus. Subsequent drug administration, lesion, and genetic manipulation experiments in laboratory animals have shown that over time, post-learning memories are transferred from the hippocampus to the cortex and stored more stably and for longer periods of time. Although this process is now known as "memory consolidation," it has been unclear when and where memories are stored at the cellular level.
Long-term potentiation of synapses (LTP)
The brain is composed of a vast number of neurons, which communicate with each other via synapses. Changes in the efficiency of synaptic transmission are called synaptic plasticity, which is considered to be a memory phenomenon at the cellular level. One of the representative phenomena of synaptic plasticity is long-term potentiation (LTP), which is a long-term enhancement of synaptic transmission efficiency (Figure 1). LTP is induced in the brain after learning (Fig. 1). However, it has been technically difficult to precisely examine the timeline in which LTP occurs after learning. For example, in drug experiments, it was difficult to inhibit LTP only at the targeted time because it continues to act at the site of administration. Therefore, we worked on the development of a new technique to obtain spatiotemporal information on when LTP occurs.
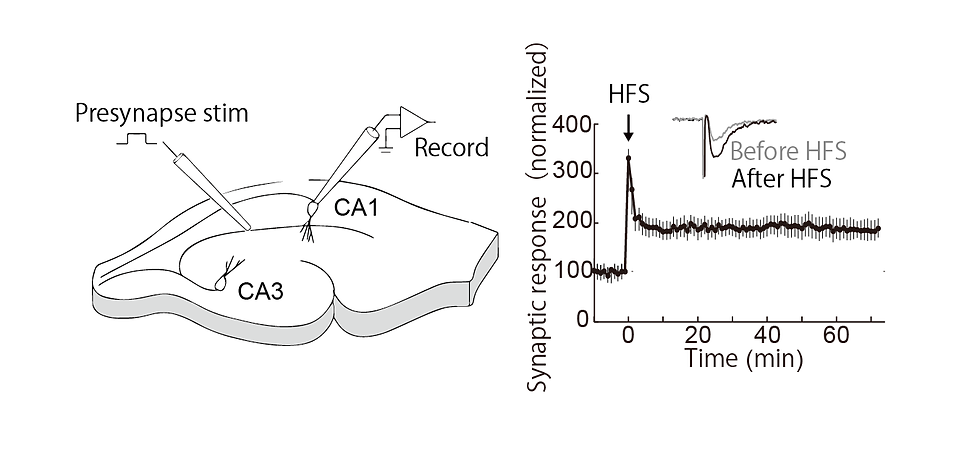
Figure 1. An example of an experiment in which long-term potentiation (LTP) was recorded from a hippocampal slice, stimulating presynaptic fibers in CA3 and observing the response in CA1. Right: High-frequency stimulation results in long-term potentiation of subsequent synaptic transmission (Goto unpublished).
Molecular Mechanisms of LTP
In the central nervous system, synapses are formed in mushroom-like structures called dendritic spines, and the spine structure is enlarged upon LTP and depends on actin polymerization. Hayashi Lab previously examined translocation of actin binding proteins in spine upon LTP induction (Bosch2014Neuron). Cofilin was originally identified as a cleavage molecule of fibrillar actin (F-actin), but it is known to be more stable at high concentrations. Hayashi Lab showed that cofilin interacts with F-actin at a nearly 1:1 density, stabilizing F-actin and thereby expanding the spine.
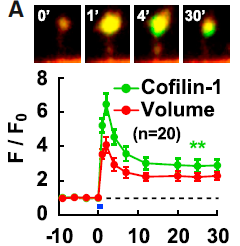
Figure 2. two-photon image of dendritic spine of hippocampal neurons coexpressing red fluorescent protein (red, indicating morphology) and cofilin-GFP (green); LTP-induced spine enlargement and simultaneous cofilin accumulation at the spine head. Bosch2014Neuron
Development of a Light-induced LTP cancellation method
Based on the above previous studies, we thought that once cofilin is inactivated, LTP can be eliminated by inactivating cofilin. superNova, a photosensitizing protein, generates reactive oxygen species (ROS) upon light irradiation and inactivates only the neighboring proteins. Using this method, called CALI (chromophore-assisted light inactivation), cofilin can be inactivated by light (Fig. 3). After coexpression of cofilin-SuperNova fusion protein and cofilin-GFP in neurons and induction of LTP, spines were irradiated with light to induce CALI. As a result, LTP cancellation was observed along with cofilin-GFP accumulation in the spine. This suggests that the binding of cofilin to F-actin is cooperative and that only the inactivation of some cofilin molecules can dissociate other cofilin molecules from F-actin.

Figure 3. LTP-induced cofilin accumulation at the spine head and its cancellation by CALI. (Left) Light irradiation of SuperNova induces the production of reactive oxygen species (ROS), which inactivate the fused cofilin. (Right) Dendritic spine of hippocampal neurons coexpressing red fluorescent protein (red, showing morphology), cofilin-GFP (green), and cofilin-SuperNova (not shown); LTP induction causes spine enlargement and cofilin accumulation at the spine head. Light irradiation induces CALI, which causes cofilin accumulation to disappear and the spine to shrink at the same time. Scale bar 1 µm
When the whole slice was then irradiated with light, only the LTP-induced spines shrank, while the non-LTP-induced spines were unaffected (Figure 4). This indicates that the effect of CALI is specific to LTP. When LTP was induced again in the shrunken spines, LTP was induced normally, indicating that LTP cancellation by CALI is a reversible response and not simply a destruction of the spines.
Also, this effect had a time frame, and there was no effect when light was irradiated before LTP induction or after 30 minutes after induction. In other words, we found that light irradiation within 30 minutes after LTP induction can cancel LTP.
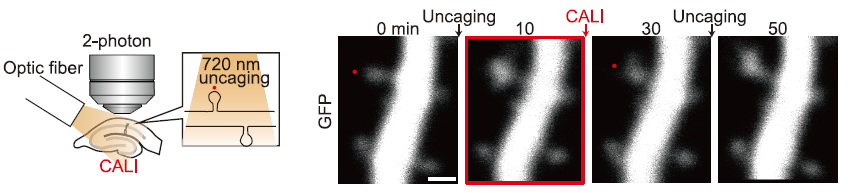
Figure 4. Figure from Goto et al., Science 2021 Fig. 1. The whole slice is irradiated by optical fiber. Red dots indicate LTP induced by uncaging.
This method is novel in the following points. First, simply by externally expressing CFL-SN and exposing it to light, it can also interfere with the function of endogenous cofilin, which is involved in LTP, and LTP cancellation can be achieved. Second, the effect of light is specific to LTP, because even if the entire cell is irradiated with light, the effect is on the spine where cofilin is accumulated by LTP (Figure 5). Finally, the effect of light can be limited to 30 minutes immediately after LTP. Since it has been difficult with existing techniques to operate immediately after LTP or limited in time, this method enables spatio-temporal analysis of LTP.
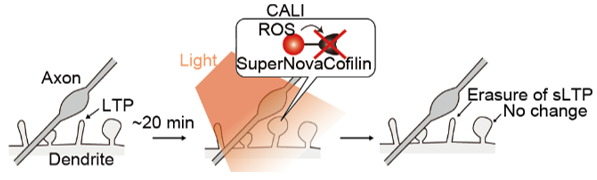
Figure 5. Specific LTP cancellation by light. The spine is enlarged by LTP and LTP is specifically cancelled by inactivating cofilin by light irradiation within 30 minutes of the enlargement.
Memory erasure by photo-inactivation of Cofilin
We examined whether memory can be erased by LTP cancellation in the living brain using the techniques described above. CFL-SN was expressed in excitatory neurons of the hippocampus by using adeno-associated virus (AAV) (Figure 6). To quantify memory, we used a learning task, the Inhibitory Avoidance test (IA test), which is performed in a box with two compartments, a dark room and a light room, to which mice can move freely. Mice prefer the dark room, so they usually move from the light room to the dark room within 30 seconds after they are placed in the light room. However, if mice are electrically shocked immediately after entering the dark room, they do not immediately enter the dark room even if they are put in the light room again on the next day. By measuring this latency to enter the darkroom, memory can be evaluated. When CALI was induced between 2 and 20 minutes after learning by the IA test, no latency was observed, indicating memory was erased. These results are consistent with the brain slice results and indicate that memory can be erased by light by specifically canceling LTP within 20 minutes in vivo. This also demonstrates that LTP is induced in the hippocampus immediately after learning, which is important for memory formation.
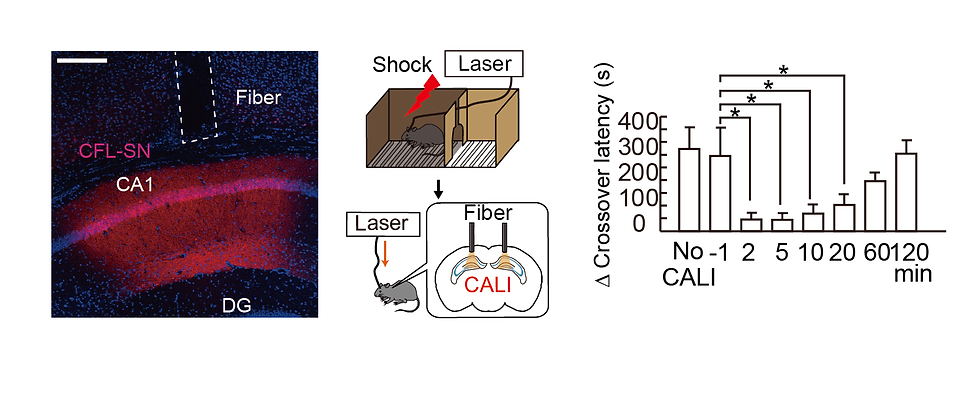
Figure 6. (Left) Image of hippocampal tissue. Red (CFL-SN expression) is observed in hippocampal CA1, and a light fiber track is observed just above. Scale bar 300mm. (Middle) Schematic diagram of IA test and CALI experiment. (Right) Light exposure 1 min before and 2, 5, 10, 20, 60 and 120 min after electrical stimulation of IA test on day 1 and observation of latency (=memory performance) on day 2.
Furthermore, we checked whether LTP cancellation erased specific memories within 20 minutes. For this purpose, IA test was performed in two boxes of different colors, shapes, and smells, Context A and Context B (Figure 7). 2 hours after learning in Context A, mice learned in Context B, and immediately after that, light was illuminated. Then, only the memory of Context B was erased. In other words, it was shown that memories formed within 20 minutes of exposure to light were specifically erased.

Figure 7. Figure from Goto et al., Science 2021 Fig2. (Left) Different memory formations in Context A and B on Day 1, and CALI is performed. (Right) Crossover latency in each box.
Synaptic Plasticity in the Hippocampus during Sleep
Although these results show that LTP is induced in the hippocampus immediately after learning, we are also interested in the subsequent role of sleep, since sleep is known to be important for memory and learning. We were also interested in the role of sleep after learning, since sleep is known to be important for memory and learning. For example, it is known that the hippocampus undergoes replay of waking neural activity during sleep, and it is assumed that this phenomenon causes synaptic plasticity again. Therefore, we investigated the possibility that LTP occurs again in the hippocampus during sleep after learning. To this end, we established our own setup for CALI by introducing a system from the Murayama Lab that illuminates light only during sleep or wakefulness (Fig. 8). We automatically detected the sleep state of mice by measuring EEG and EMG, and irradiated light to the hippocampus only when the mice were asleep or awake for 20 minutes. When CALI was performed during sleep on the same day as the IA test electrical shock, memory was impaired on the following day. On the other hand, CALI during wakefulness showed no effect. In addition, there was no effect when CALI was performed the day after the electrical shock of the IA test. These results indicate that LTP is induced in the hippocampus not only immediately after learning but also during subsequent sleep. These results indicate that memory is formed when two stages of LTP occur in the hippocampus, one immediately after learning and the other during sleep on the same day. We call the LTP that occurs immediately after learning "online LTP" and the LTP that occurs during subsequent sleep "offline LTP.
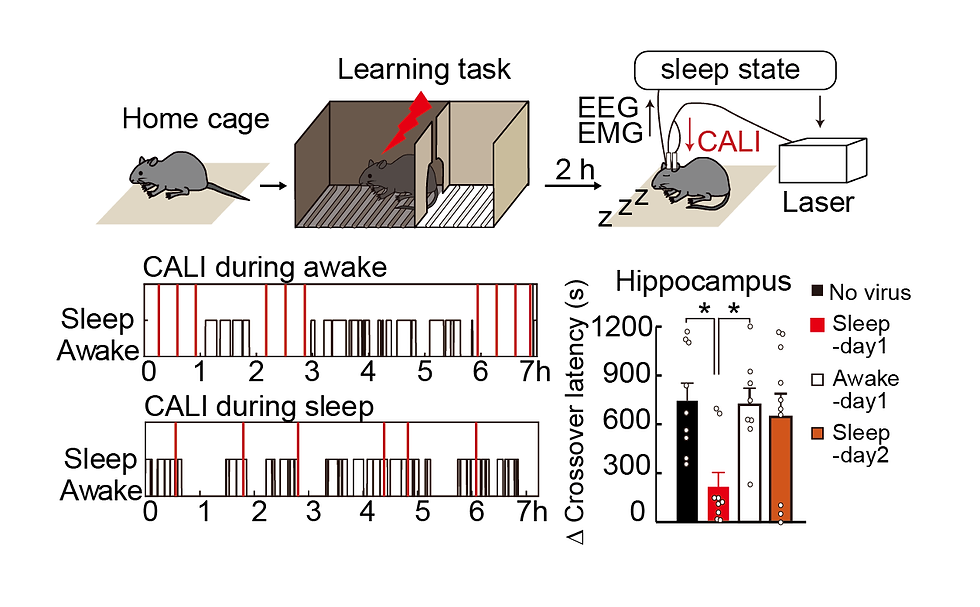
Figure 8. Schematic diagram and example of light irradiation experiment during sleep after learning. The black line indicates the sleep state, and red indicates the time point at which light was irradiated. Examples of light irradiation only during wakefulness (top) and only during sleep (bottom) are shown. Bar graphs show memory performance one day after light illumination.
Neural Activity formed by Online LTP and Offline LTP
The above results indicate that both online and offline LTP are necessary for memory in the hippocampus, but what are the functional differences between the two? Therefore, we investigated what new neural activity is formed when memory is formed by LTP. For this purpose, we used a small head-mounteed microscope, nVista from Inscopix (Fig. 9). nVista is equipped with LED, CCD, and other optics, but its total weight is about 2 g, so calcium imaging can be performed while it is mounted on the head of free-moving mice. We performed calcium imaging before and after learning from mice expressing CFL-SN and GCaMP6f in hippocampal CA1. CALI was performed 2 minutes after electrical shock in the group with online LTP cancellation and 2 hours after electrical shock in the group with offline LTP cancellation.
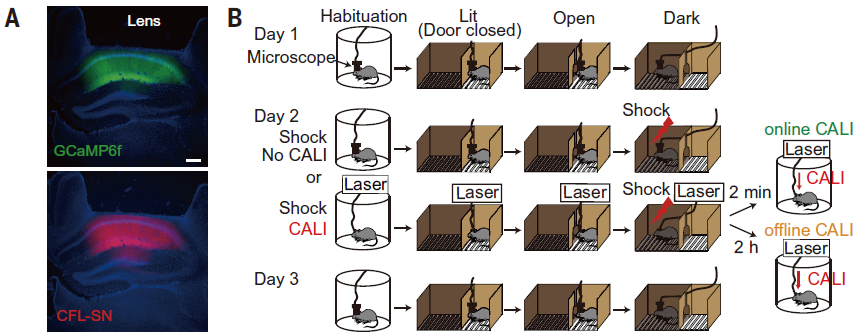
Figure 9. A. AAV9-CAG-DIO-CFL-SN-P2A-GCaMP6f injection into CaMKII-Cre mice. B. Experimental design. (Goto et al., Science 2021 Fig4).
First, to detect activity changes in response to the learned space (IA test), we calculated the ratio of neural activity in the learning environment (IA test box) to the rest of the environment (cylinder box).
We found that in control mice without CALI, cells firing more selectively in the IA test box increased after learning (Figure 10). In contrast, there was no increase in selectivity for the learning space after online LTP cancellation. Offline LTP cancellation had no effect on selectivity for the learning space. In conclusion, we found that online LTP immediately after learning increased the selectivity of neural activity in the learning space.
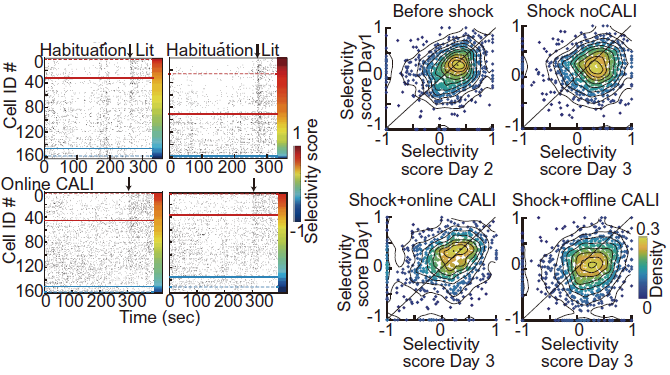
Figure 10. (Left) Raster plots of Habituation (cylindrical cage) and IA test cells in the light chamber. Cells above the red line are specifically firing in the light chamber and below the blue line are specifically firing in the cylindrical cage. In controls, the number of cells specifically firing in the light chamber increases after learning, but is suppressed after online LTP cancellation. (Right) Distribution density of cells based on selectivity score. In the group without CALI, the number of cells with a higher selectivity score increases on Day 3, but this is suppressed after online LTP cancellation. The figures are modified from Goto et al., Science 2021 Fig. 4.
So what is the role of offline LTP during sleep? It is believed that the activity of cells responsible for memory is synchronous and coordinated. Therefore, we performed PCA analysis of calcium traces to detect synchronous firing before and after learning. When PCA was performed on the calcium traces of the detected cell groups, we could see synchronous firing at the time points detected as large deviations (Figure 11F,G). When PCA is applied to the calcium traces before (Day 1) and after (Day 3) learning, large deviations can be seen after learning, indicating an increase in synchronous firing after learning. We also counted the number of cells firing in each frame and found that the number of frames with more cells firing simultaneously increased after learning (Figure 11H). Both with online LTP cancellation and with offline LTP cancellation, there was no increase in synchronous firing. In other words, we found that synchronous firing was acquired by online LTP immediately after learning and by offline LTP during subsequent sleep. We also observed synchronous firing of cells while peering into a dark room and backing away during the IA task, i.e., while recalling memories of electrical shock (Figure 11I). This suggests that synchronous firing cells are involved in memory.
We also confirmed that cells that show specific firing in the learning space after learning are more likely to acquire synchronous firing properties. This suggests that hippocampal cells become specific to the learning space by online LTP, and then acquire more synchronous firing by offline LTP during sleep.
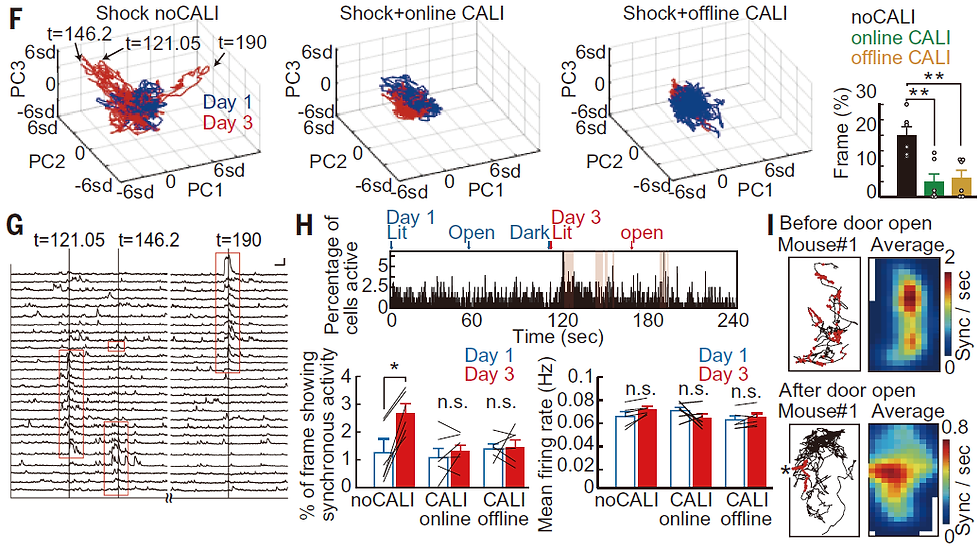
Figure 11. From Goto et al., Science 2021 Fig4. See text for details.
Synaptic Plasticity in the Cortex
Next, we examined the time and brain regions where memory is transferred to the cortex. We focused on a region of the cortex called the anterior cingulate cortex (ACC). This region is known to be activated during recall of old memories (weeks in the case of the mouse experiment). Inactivation of ACC impaired recall of old memories while it had no effect on recall of new memories. Therefore, we used AAV to express CFL-SN only in excitatory neurons in the anterior cingulate cortex and performed the same CALI experiment as in the hippocampus (Fig. 12A). Light irradiation immediately after learning had no effect on memory performance, unlike in the hippocampus (Figure 12B). Furthermore, light exposure every 20 minutes for 8 hours after learning had no effect, indicating that, unlike the hippocampus, LTP was not induced in the anterior cingulate cortex at the time of learning (Figure 12C). Furthermore, when CALI was performed during sleep the day after learning, the memory was erased (Figure 12D,F). This effect was no longer observed on the 25th day of learning (Figure 12E). This indicates that LTP is induced in the anterior cingulate cortex during sleep on the day following learning. Based on previous studies, it was thought that in the anterior cingulate cortex, cells responsible for memory are tagged after learning (also called silent engram cells) and then gradually become responsible for learning over time. The results of this study suggest that the induction of synaptic plasticity in the anterior cingulate cortex during sleep on the day after learning is important for memory and for the subsequent formation of long-term memory in the cortex.
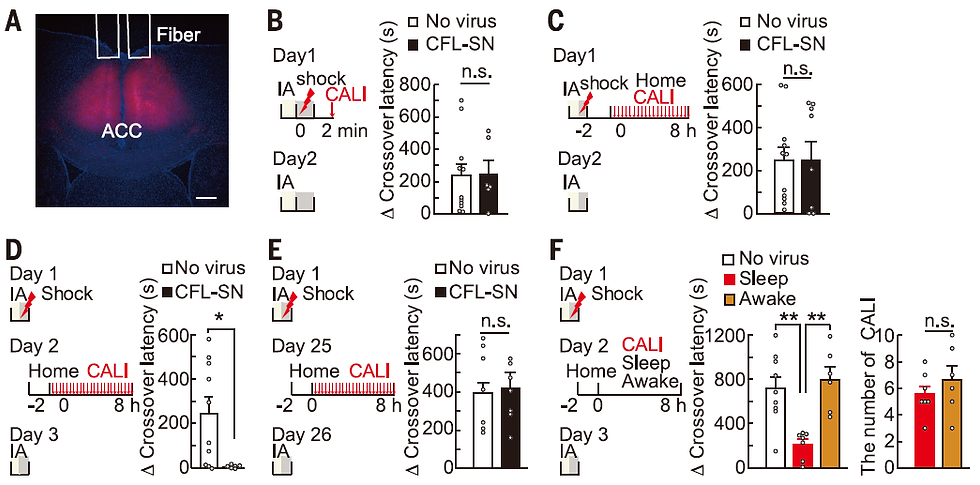
Figure 12. From Goto et al., Science 2021 Fig5. See text for details.
Summary and Future Prospects
These results show that memory is formed in the hippocampus by LTP immediately after learning (online LTP) and during sleep that day (offline LTP), and another LTP event is further induced in the anterior cingulate cortex during sleep the following day (Figure 13). These results represent a new spatiotemporal model of memory consolidation based on LTP, a cellular-level memory.
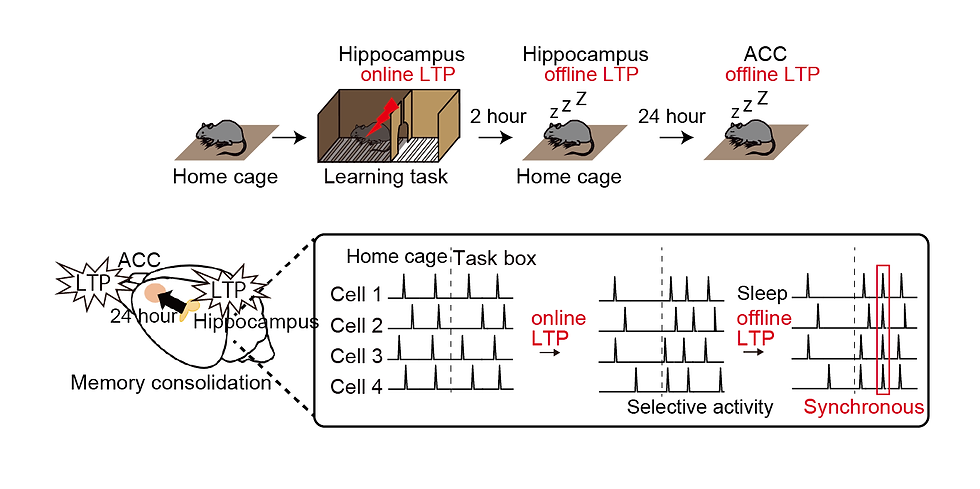
Figure 13. time frames of LTP in the hippocampus and anterior cingulate cortex (top). Cellular activity acquired by online LTP and offline LTP in the hippocampus (bottom).
Since NMDA receptor-dependent LTP is a common mechanism not only in the hippocampus and anterior cingulate cortex but also in many brain regions involved in memory, this techniques that uses light to cancel NMDA receptor-dependent LTP can detect the time frame of LTP in multiple brain regions involved in memory. Combined with calcium imaging, it is also possible to analyze in detail the activity of cells (engrams) formed by that LTP.